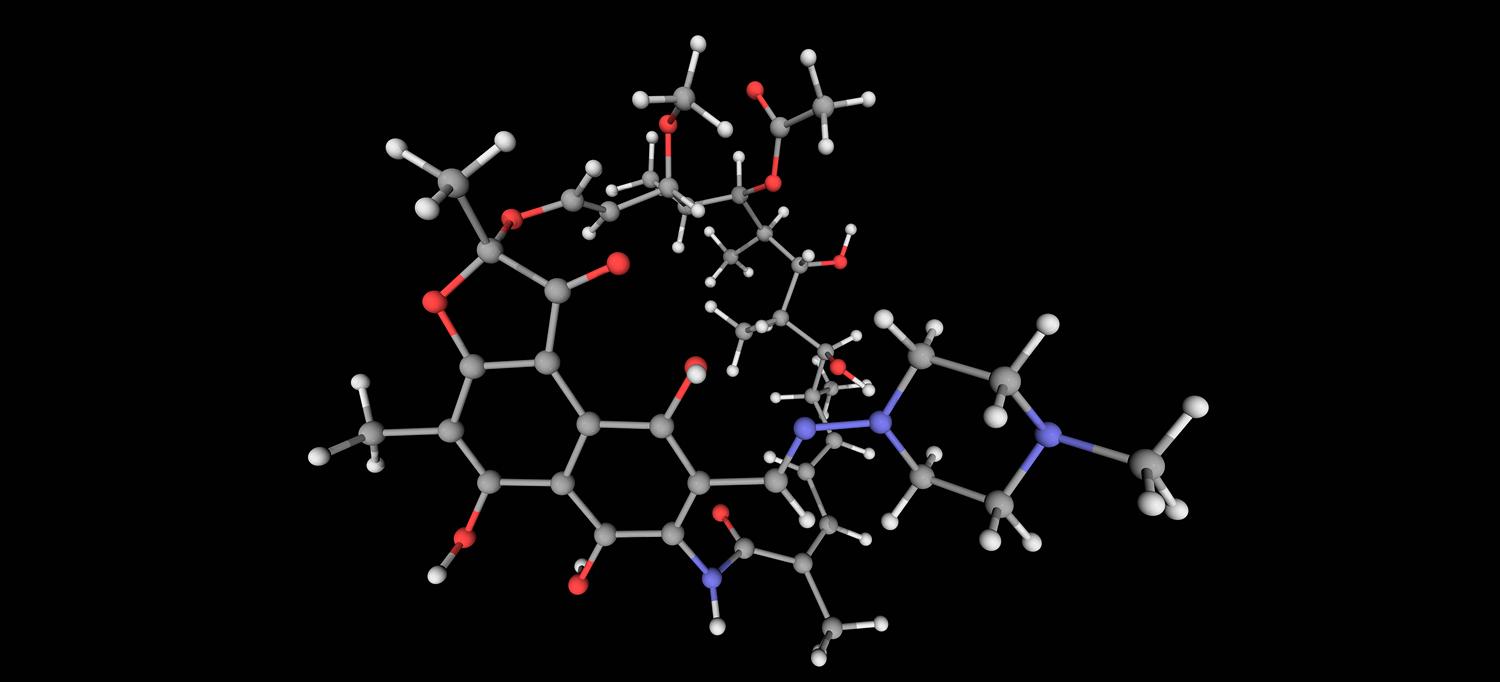
Photo: LAGUNA DESIGN/Getty
Modern medicine depends on antibiotics to treat infections by disabling targets inside bacterial cells. Once inside these cells, antibiotics bind to certain sites on specific enzyme targets to stop bacterial growth. Randomly occurring changes (mutations) in the genes for these targets occur naturally, in some cases making the target harder for the antibiotic to attach to and that bacterial version resistant to treatment.
For this reason, the more antibiotics have been used over time, the greater the chances that bacterial populations will evolve to have mutants resistant to existing antibiotics, and the more urgent the call for new approaches to keep the treatments from becoming obsolete. Researchers have for decades studied resistant mutants in hopes that related mechanisms would guide the design of new treatments to overcome resistance. The effort has been limited, however, because naturally occurring resistant mutants represent a small fraction of the mutations that could possibly occur (the complete mutational space), with most drug binding site mutations to date having been overlooked.
To address this challenge, a new study led by researchers at NYU Grossman School of Medicine applied a technology called MAGE (multiplex automated genome engineering) to generate the full inventory of mutations in the bacterial species Escherichia coli where the antibiotic rifampicin attaches to and disables an essential bacterial enzyme known as RNA polymerase (RNAP). The study authors created 760 unique RNAP mutants by replacing each of the 38 amino acid building blocks that make up the rifampicin binding site on E. coli with each of the 20 amino acid options present in nature. Growth of this mutant pool was then tested under different conditions, including treatment with rifampicin.
Published online August 30 in the journal Nature, the study found two mutants, L521Y and T525D, that are hypersensitive to rifampicin. Not only does the antibiotic prevent these mutants from growing, it nearly obliterates the mutant bacterial populations. This is a remarkable finding, say the authors, because rifampicin normally does not kill E. coli, or many other bacterial pathogens, but only stops their growth.
“This work provides a map of antibiotic–bacterial RNAP interactions that will be of value to chemists working to build on the study effects by changing, not bacterial binding site residues, but instead the structure of rifampicin and other antibiotics so that they bind tighter for increased potency,” said study co-senior investigator Evgeny A. Nudler, PhD, the Julie Wilson Anderson Professor of Biochemistry in the Department of Biochemistry and Molecular Pharmacology at NYU Langone Health. “Our findings also suggest ways of improving rifampicin’s ability to bind to proteobacteria, actinobacter, and firmicutes, bacterial groups that include natural RNAP mutations that render them vulnerable to rifampicin.”
How Rifampicin Kills Bacteria
E. coli stores genetic instructions in DNA chains, but then converts them into a related genetic material in RNA, with RNAP building the RNA chains that guide the building of proteins out of amino acids. The mutants created in the new study revealed that rifampicin kills bacteria by stalling RNAP, and so causing collisions between it and cellular machinery that operates in the same molecular space to duplicate DNA as cells divide and multiply. This in turn causes lethal breaks in both strands of bacterial DNA.
In other insights from the study, some of the E. coli RNAP binding site mutations were found to greatly increase the speed with which RNAP builds RNA, and so the speed that it uses up raw materials, including nucleotide building blocks like pyrimidines. The work has significant implications, say the researchers, for the understanding of the mechanism of action used by nucleotide analogues like the anti-cancer drug 5FU. Understanding how nucleotide depletion sensitizes cells to nucleotide supplies may help in the design of new combination therapies, they say.
“These techniques could be applied to map the binding sites of other drug types, and especially to those vulnerable to resistance,” said co-senior study investigator, Aviram Rasouly, PhD, a research scientist at NYU Langone.
Funding support for the study was provided through National Institutes of Health grants T32 AI007180 and R01GM126891 and the Blavatnik Family Foundation. The study was led by MD/PhD student Kevin Yang. Other NYU Langone researchers involved in this study were Maria Cameranesi, Criseyda Martinez, Manjunath Gowder, Yosef Shamovsky, Vitaliy N. Epshtein, Khaled Alzoubi, Zhitai Hao, and Ilya Shamovsky. Dr. Nudler is also an investigator with the Howard Hughes Medical Institute.
Media Inquiries
Greg Williams
Phone: 212-404-3500
Gregory.Williams@NYULangone.org